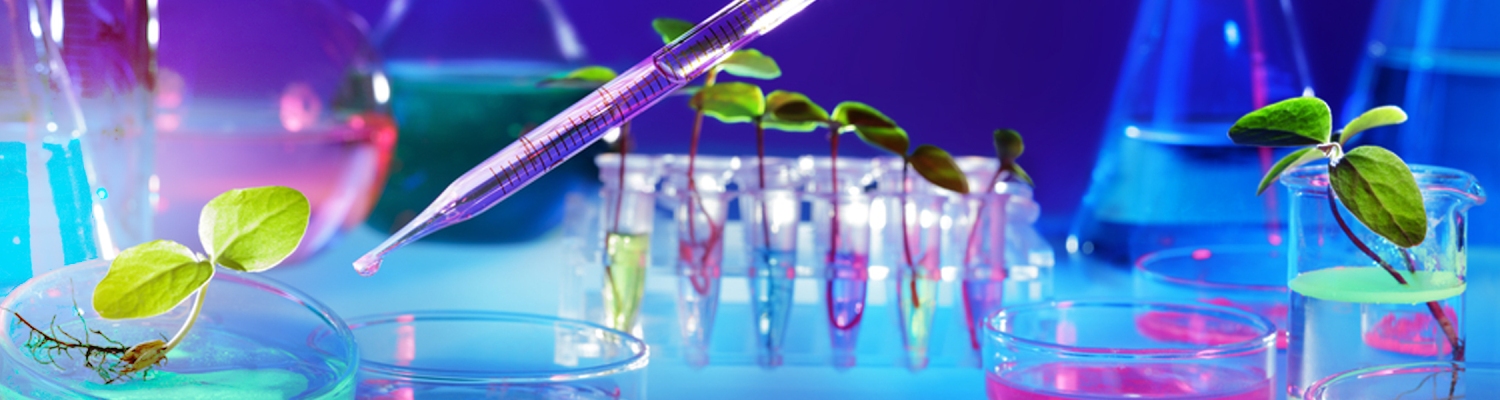
Krystyna Niesiobedzka*
Received: March 31, 2023; Published: April 06, 2023
*Corresponding author: Krystyna Niesiobedzka, Warsaw University of Technology, Faculty of Building Installations, Hydrotechnics and Environmental Engineering, Poland
DOI: 10.32474/CTBM.2023.03.000167
The aim of the study was to investigate and compare the heavy metals (Cu, Pb and Zn) concentrations in Festuca rubra of three groups of grassy ecosystems with different anthropopressive risk (A ecosystem - urban soils, B ecosystem - rural soils, C ecosystem - roadside soils). The strongest pollution was recorded for grass of A and C ecosystems. They were caused mainly by the presence of Pb which their concentrations significantly exceeded critical values for plants confirming unequivocally the inflow of metals from anthropogenic sources typical for areas with a high degree of industrialization and sources related to the automotive industry in A and C ecosystems. The concentrations of this metals fluctuated at the level of natural concentrations in plant B ecosystem indicating the lowest bioaccumulation. The average values of Cu, Pb and Zn the above-ground part of in the grass changed in the following order:
a) Cu: A ecosystem - 11.0 mg.kg-1 > C ecosystem -10.3 mg.kg-1 > B ecosystem - 6.5 mg.kg-1
b) Pb: A ecosystem - 95.2 mg.kg-1 > C ecosystem- 35.1 mg.kg-1 > B ecosystem - 7.7 mg.kg-1
c) Zn: A ecosystem - 133.0 mg.kg-1 > B ecosystem - 97.1 mg.kg-1 > C ecosystem - 27.0 mg.kg-1
Keywords: Festuca rubra; cu; pb; Zn; bioaccumulation
The condition of the vegetation, its development and health reflect the ecological conditions in a given area. Abnormal growth of a large plant population in a given area is usually a manifestation of anthropogenic environmental degradation. Plants react quickly to chemical changes in the environment. In biological monitoring, various plant species are used, which are characterized by a high accumulation capacity of metals in tissues. Monitoring the contamination of heavy metals forage from meadows and pastures is an extremely important issue. Undertaking this type of research is advisable not only for cognitive reasons, but also for utilitarian reasons. As a result of the progressing industrialization, changes in the chemical composition of the environment are taking place, leading to the disturbance of the homeostasis of “soil and plant ecosystems” [1-3]. The problem of excessive accumulation of heavy metals concerns mainly grass communities in the conditions of urban agglomerations and in the vicinity of communication routes. In response to environmental pollution or its strong transformations, the so-called synanthropic vegetation [4-6], which include mainly Hordeum murinum, Bromus sterilis, Eragrostis minor, Puccinellia distance (the latter occurring in large numbers along communication routes) [7].
In most Polish cities, Festuca rubra, Lolium perenne and Poa pratensis account for a significant share in the coverage of street lawns [8]. In this study, the subject of research was the grass (Fes tuca rubra), which is characterized by a slow growth rate and high tolerance to frost and drought. It has narrow and delicate leaf blades. It creates loose low tufts and, as a result, an even, dense, moderately compact turf. In Poland, it is quite common and inhabits mainly roads, ditches, embankments, meadows, slopes and ruderal places. The species tolerates unfavorable weather conditions as well as temporary drought and long-term flooding (e.g., due to long-lasting rains) [9]. The main source of heavy metals for plants is the soil from which they are taken up by the root system. Significant amounts of some elements can also be absorbed by plants from atmospheric dust and rainfall through leaf blades [10]. All substances present in excessive amounts in the environment may create stressful conditions for plant development. Some of them are particularly active and harmful due to their specific role in biochemical processes and their mutual interactions (synergistic or antagonistic) [11]. Plants take up copper both in an active way (related to metabolic processes) and passively along with the transpiration water flow [12-13]. The tolerance of plants to excess copper is generally high, but excessive amounts of it cause disturbances in metabolism and, as a result, limit growth and development.
Copper binds proteins strongly and is deposited in the intercellular spaces. Lower plant organisms sometimes deposit excess copper in the form of copper sulphide CuS on the surface of cell membranes. Lead uptake is a passive process dependent on the concentration of soluble forms in the soil solution, which is influenced by such factors as: pH of the solution, soil organic matter content, activity of bacterial flora, redox potential. An important barrier to lead transport in plant tissues is the root surface, where a significant amount of lead is retained and bound by carboxyl groups of pectin compounds present in the mucus covering the root surface. The lead taken up by the roots is accumulated in them, and the degree of transport to the green parts of the plant is very limited (Kabata- Pendias and Pendias, 1999). At a relatively high concentration of lead in the soil solution, it can get to the leaves with the transpiration current through conductive bundles [14]. The greatest amount of lead deposits is found in the cells that build the leaf bundle and in the root cell membranes, disrupting its proper development [15]. Lead taken up by the root reaches the above-ground parts of plants only in negligible amounts. Rainfall lead can also be transported to the leaves through stomata or after previous cuticle damage, e.g., by acid rain or ozone. The soluble forms of zinc are easily absorbed by the root system of plants depending on the type, species or variety of the plant.
Soil conditions also have a significant impact on this process, in particular soil pH and the ratio of calcium ions to zinc Ca2+ / Zn2+ ions in the soil solution. Anthropogenic zinc (like copper) is more easily absorbed by plants than the same element derived from its natural resources in the soil. Plant tolerance to high concentrations of zinc is quite common and plants may not show toxic symptoms even in the event of significant bioaccumulation. The nature and composition of plant and soil pollution are closely related to the quality of the atmosphere. Dust pollutants with metals present in them settle on the surface of plants as a result of dry deposition, which, under favorable conditions, can penetrate into the internal tissues of plants [16], causing their excessive bioaccumulation. The values of average (normal) and critical metal content for plant growth are summarized in Table 1 [17]. Critical metal content is the content that causes a decrease in the rate or inhibition of shoot growth, diseases and discoloration as well as premature aging, a deficiency of macro- and micronutrients in the plant [18,19] studied five species of plants that dominate in lawn plant communities, including red fescue (Festuca rubra), perennial ryegrass (Lolium perenne L.) and meadow grass (Poa pratensis), in which it determined the content of trace elements (Cu, Pb, Zn, Ni, Cd).
In the mentioned plant species, the contents of Cu, Pb and Zn oscillated successively at the level of 3.3; 6.7; 6.8 mg.kg-1 (Festuca rubra), 1.6; 6.2; 6.3 mg.kg-1 (Lolium perenne L.) and 3.4; 6.0; 6.0 mg.kg-1 (Poa pratensis). There was no significant difference in the content of individual elements between the grass species. According to [20] all these plant species show great phytoremediation abilities in relation to the tested metals. Plant nutrition in soil conditions takes place in a close relationship that has been formed between two systems: roots (bio colloids) and soil colloids [21]. Each of these systems is capable of exchanging cations with a different colloidal system or with a soil solution. The direction of exchange depends on the electric potential and the degree of saturation of the colloids with cations in a given system.
The main factors influencing the root absorption of metal ions from the soil solution by plant roots include:
a) Concentration of ions in the solution: for small concentrations (<1miligramequivalent dm-3) the value absorption is proportional to the concentration of ions in the water phase of soil.
b) Chemical properties of ions (lower charge ions are generally easier absorbed).
c) The influence of pH and Eh on the precipitation process of some ions.
d) Ion interactions (antagonism and competition for sorption in the complex soil sorption or ionic absorption by plants) [22].
e) Type and number of cations in the sorption complex.
f) The degree of saturation of plant plasma biocolloids with cations and the processes of assimilation and dissimilation as processes regulating the life energy of a living organism [23].
The mechanism of the uptake of elements by the roots is a complex process, which consists of: rhizosphere processes, cell transport and cation exchange through cell membranes. For plants, it is not so much the total content of elements in the soil that matters as the proportion that can be taken up by them from the soil. This part is referred to as the available (bioavailable) form and usually constitutes a certain percentage of the total content of the element in the soil, depending on many factors, including the physicochemical properties of the soil and the original form of metals in which they entered the soil ecosystem. Plants are an important link in the movement of metals from soil into animals and humans. Due to the participation in the diet of consumed plant products, they are considered an important source of heavy metals. The aim of the study was to investigate and compare the heavy metals (Cu, Pb and Zn) concentrations in Festuca rubra of three groups of grasssy ecosystems with different anthropopressive risk (A ecosystem - urban soils, B ecosystem - rural soils, C ecosystem - roadside soils). This work should give an answer to the question: „Can Festuca rubra be used as a bioindicator”?
The research covered the above-ground part of grasses from three groups of grassy ecosystems located in Poland. The selection of research areas was guided by their syntaxonomic similarity, while maintaining a clear division in terms of their location (A - urban grassland ecosystem, B - rural ecosystem, C - roadsides of communication routes). The dominant species of grass in the collected samples of the plant material were: Festuca rubra (occurring mainly in the form of a clump - Festuca rubra subsp. Comutata), Lolium perenne and Poa pratensis. The samples of the above-ground parts of the grass were collected during the period of increased plant vegetation (May - June) (Figure 1).
a) Ecosystem - covered a selected area of the Warsaw agglomeration, exposed to the impact of various sources of pollution (industrial and automotive emissions), but without particular dominance of communication sources.
b) Ecosystem - these were areas of agricultural land, no longer covered by agrotechnical measures and excluded from agriculture (wasteland) and post-agricultural land left without human interference (fallow land). Many important ecological aspects of the natural vegetation of these plots are still poorly understood.
c) Ecosystem - areas along three communication routes of varying intensity: national road E77 and two voivodeship roads - DW618 and DW689. The samples were collected at a distance of 5-10 m from the edge of the road.
The above-ground part of the plant (grass) intended for research was cut right next to the ground. Three samples of grass were taken each time from an area of 1 m2, then mixed and, after averaging, analyzed after previous preparation. Festuca rubra subsp. Was selected from the collected plant material. comutata and this species was submitted for analysis. The collected plant material was washed with distilled water, dried in an oven at 65°C, and then homogenized. After homogenization and weighing, the grass samples were analyzed according to the procedures specified by [24].
The total concentrations of Cu, Pb and Zn were determined by flame atomic absorption spectrometry (AAS). 1-gram samples of plants were subjected to wet mineralization with the use of a mixture of acids: nitric (V) and chloric (VII) in a volume ratio of 3: 1. A 100 mm torch was used and was fed with a stoichiometric mixture of air and medical acetylene (acetylene A). The flame temperature was 2100-23000 C. Philips hollow cathode lamps were used as radiation sources. The spectrophotometer was controlled by Unicam’s Atomic Absorption - Data Station 1.7 software by Unicam. The detection limits of copper, lead and zinc in terms of mg.kg-1 of soil dry weight were respectively: Cu (0.03), Pb (0.05) and Zn (0.01).
The concentrations of copper, lead and zinc in the aboveground part of the grass are shown in Figure 2. The obtained results of the research for individual crops (ecosystems A, B and C) are presented in Figures 3-5. The average concentration of copper in the above-ground part of the plant samples was respectively: 11.0 mg.kg-1 in samples representing the group of A ecosystem, 6.5 mg.kg-1 - in samples of B ecosystem and 10.3 mg.kg-1 in samples of C ecosystem, and concentration ranges: 6.3-17.9; 1.2-16.6 and 1.0- 34.4 mg.kg-1 (Figures 2 & 3). The highest value of the median copper concentration was related to the grass samples of an ecosystem (16.1 mg.kg-1), the smallest - to the grass samples of B ecosystem (5.3 mg.kg-1), while in the grass samples of C ecosystem the median copper concentration reached the value of 7.1 mg.kg-1 (Figure 3). In this group, the highest concentration of copper was also recorded, amounting to 34.4 mg.kg-1. Concentrations of copper in samples of grasses collected along the E-77 route significantly exceeded the natural level (3–15 mg.kg-1), as well as the content critical for plant growth, ranging from 15 to 20 mg.kg-1. The plants collected on the sections of the remaining roads did not show excessive accumulation of copper.
Figure 2: Cu, Pb and Zn concentrations in the above-ground part of the grass in three groups of ecosystems (average, min and max values).
In the grass samples from the area of the Warsaw agglomeration, no increased content of this element was found, except for two samples for which the concentration values (17.9 and 16.9 mg.kg-1) only slightly exceeded the natural level. The natural lead content in plants ranges from 1 to 5 mg.kg-1 and the critical for plant growth 10-20 mg.kg-1. The average lead concentrations in the grass samples were respectively: 95.2 mg.kg-1 (A ecosystem), 7.7 mg.kg-1 (B ecosystem) and 35.1 mg.kg-1 (C ecosystem) and median: 104.2; 6.0 and 35.1 mg.kg-1 (Figures 2 & 4). The plant of an ecosystem showed the highest variability (17.3-250.1 mg.kg-1), while the smallest – the grass of B ecosystem (4.0-18.3 mg.kg-1), while the range of variability for plants growing on roadside soils was 2.9-116.9 mg.kg-1. In most cases, lead concentrations significantly exceeded the levels of the natural content and the critical content even several dozen times and concerned the grasses of A and C ecosystems. The average concentrations of zinc in the above-ground parts of the grasses of A , B and C ecosystems were respectively: 133.0; 27.0 and 97.1 mg.kg-1 with a median of 132.5; 15.7 and 79.5 mg.kg-1 (Figures 2 & 5). In the case of the group of A ecosystem, only three samples of grasses showed higher values of zinc concentrations, exceeding the natural level (15 to 150 mg.kg-1), similarly to the grasses of C ecosystem, among which also three samples were characterized by higher bioaccumulation of zinc.
The concentrations of metals in the above-ground part of the grass changed in the following order:
a) Cu: A ecosystem (11.0 mg.kg-1)> C ecosystem (10.3 mg.kg-1) >B ecosystem (6.5 mg.kg-1).
b) Pb: A ecosystem (95.2 mg.kg-1)> C ecosystem (35.1 mg.kg-1) > B ecosystem (7.7 mg.kg-1).
c) Zn: A ecosystem (133.0 mg.kg-1)>B ecosystem (97.1 mg.kg-1)>C ecosystem (27.0 mg.kg-1).
The highest concentrations of Cu, Pb and Zn in the aboveground part of the grass (in most cases exceeding the natural level) were recorded in samples representing an ecosystem, and the lowest - in B ecosystem. The highest concentrations of copper were found in the samples of grass collected along the E-77 route, which significantly exceeded the natural level (3–15 mg.kg-1), as well the content critical for plant growth. The grass samples collected on the sections of the remaining roads did not show excessive bioaccumulation of copper. In the grasses of A and B ecosystems, no increased levels of this element were found, except for two samples for which the concentration values only slightly exceeded the natural level. The average values of metal concentrations in ecosystems A and C were high and significantly exceeded the analogous parameters in roadside wild grasses on the Qinghai-Tibet plateau [25,26] also noted high concentrations of metals in grasses (Cu - 6.02-35.50 mg.kg-1, Pb - 6.26-16.56 mg.kg-1, Zn - 33.57-138.60 mg.kg-1), often exceeding the critical concentration levels of metals proposed by IUNG. The limit values of metal concentrations in plants for fodder purposes are as follows: Cu ≤ 25 mg.kg-1, Pb ≤ 10 mg.kg-1 and Zn ≤ 100 mg.kg-1.
Comparing the results obtained in this study with the suggested values by IUNG, it can be concluded that copper concentrations in grasses did not exceed the limit values in any object, while lead was present in large amounts, almost 10 times higher in an ecosys tem and over 3 times higher in C ecosystem. Lead bioaccumulation in the grasses of B ecosystem did not exceed the limit values. Excess amounts of zinc were found only in the grass of an ecosystem, the average concentration of which exceeded the limit value by 32.97 mg.kg-1. In the grass samples of Torun, the concentrations of Cu, Pb and Zn were as follows: Cu-20.77, Pb-21.39, Zn-79.12 mg.kg-1 and were strongly correlated with total metal concentrations in soils (for Cu: R-0.7335, for Pb: R-0.9520 and for Zn: R-0.4664) [27]. Lead concentrations in the plants of the studied ecosystems were significantly higher than in the grasses studied by [28] (with an average content of 6.7 mg.kg-1). The diagram organizing the total concentrations of Cu, Pb and Zn in the plant material indicates a significant bioaccumulation of metals in A and C ecosystems (urban area and roadsides of communication routes). This fact confirms the significant impact of Anthrop pressure on the plant of A and C ecosystems. The plant of B ecosystem indicated ecologically clean areas of Poland, but in several cases excessive zinc content was found. The excessive amount of zinc in festuca rubra on rural areas (grazing livestock) may pose a risk of this metal entering the trophic chain.
To check whether the objects selected for the study show statistically significant differences in the concentrations of Cu, Pb and Zn in the above-ground part of plants between ecosystem groups A, B and C, the method of the Least Significant Differences NIR (LSD least significant difference test) was used. The test results are presented in Table 2, specifying for which variables their differences between ecosystem groups A, B and C are statistically significant, taking into account the p values.
The test showed that the total concentrations of Pb and Zn in plants showed statistically significant differences between the groups of ecosystems A-B, B-C and A-C, while the differences in Cu concentrations were statistically insignificant. The test results confirmed statistically significant differences in Pb concentrations in plants in relation to the compared groups A-B, B-C and A-C. t Zn concentrations significantly differed between groups A-B and B-C. The lack of significant differences between A and C ecosystems may indicate a similar nature of atheroprogression to which these areas are subject. The research showed significant differences between the concentrations of metals in plant samples from three groups of grassy ecosystems, confirming the influence of anthropogenic sources of metals on grass along communication routes and in the area of urban agglomeration. The plant species - Festuca rubra can be used as a bioindicator to assess the degree of environmental pollution.
Bio chemistry
University of Texas Medical Branch, USADepartment of Criminal Justice
Liberty University, USADepartment of Psychiatry
University of Kentucky, USADepartment of Medicine
Gally International Biomedical Research & Consulting LLC, USADepartment of Urbanisation and Agricultural
Montreal university, USAOral & Maxillofacial Pathology
New York University, USAGastroenterology and Hepatology
University of Alabama, UKDepartment of Medicine
Universities of Bradford, UKOncology
Circulogene Theranostics, EnglandRadiation Chemistry
National University of Mexico, USAAnalytical Chemistry
Wentworth Institute of Technology, USAMinimally Invasive Surgery
Mercer University school of Medicine, USAPediatric Dentistry
University of Athens , GreeceThe annual scholar awards from Lupine Publishers honor a selected number Read More...